Development of the Heart Morphogenetic Field in the Axolotl Embryo
Introduction
There are two modes of development common to most species in the animal kingdom.Virtually all embryos undergo both mosaic and regulative development at some point during their growth. Regulative development generally occurs in early gastrulation when cells are induced to form different structures according to the cell-cell signaling interactions in a specific area of the embryo that lead to the conditional specification of a cell's fate. A cell undergoing regulative development can be transplanted to another part of the embryo and form whatever structure belongs in that area instead of the structure that it would have originally formed because it is competent to receive the different signals from the new cells around it.
The second mode of development is mosaic development. Mosaic development results from the autonomous specification of a cell's fate. These cells, instead of depending on cell-cell interactions, are determined by cytoplasmic factors contained within the cell itself. These cells will form a given structure even if they are moved to a new location and are exposed to cell-cell interactions and signals that differ from their original position. Most organisms contain tissues which may undergo one or both of these developmental mechanisms at a given time (Gilbert, 2003).
The developmental pattern of the amphibian heart will be studied by bisecting the heart primordial field and observing the subsequent development of the heart, if present. If the heart were to develop using an autonomous mechanism, the cells would develop normally even if the two sides were not in physical contact with one another. This would lead to half a heart growing on either side of the incision. However, if instead the heart developed using a regulative mechanism, then the cells on the two sides would compensate for the perceived absence of the other cells. This would lead to the development of two distinct, and perhaps functional, hearts (Hamburger, 1960).
The heart morphogenetic field was split using two different techniques. First the field was split using aluminum foil. This bisection ensures that an impenetrable barrier exists between the two primordia and cuts off all signaling between cells on opposite sides of the foil. However, given that this material is completely unnatural to the embryo, this implantation technique could be excessively stressful to the embryos. Thus a second technique was modeled after the Hamburger experiment for the formation of two hearts, and involved the bisection of the heart morphogenetic field of using a transplant of non-heart tissue from older embryos. These tissues would serve as a barrier to the intracellular signals and already be specified as some other tissue. Therefore the grafts would be impervious to the heart signals. Since these transplants would be of axolotl tissue as opposed to some foreign source, such bisection would be less stressful and would presumably heal faster than bisection using foil.
Foil technique
1. Prepare microtools for surgery.
2. Prepare 1% agarose-coated operating dish; rinse with 100% HBSt and antibiotics.
3. Autoclave a small piece of aluminum foil; this foil will be used to bisect the morphogenetic fields of the heart.
4. Sort embryos by age, stop development of stage 15 axolotl embryo by placing in 4°C. The developing embryo is sensitive to temperature and will develop faster at higher temperatures (Hamburger, 1960).
5. Manually dejelly embryo in Steinbergs solution as demonstrated in video on next page. Use fine forceps to break hole in the hard jelly coat. Maneuver embryo out. Be careful not to puncture the embryo!
6. Transfer embryos into well of operating dish containing increasing concentrations of HBSt and antibiotics. Remove half the solution with pipetteman and fill with 100% HBSt. After a minute remove half the solution and fill with 100% HBSt. Allow embryo to sit 2 minutes in high salt solution. This will cause the vitelline membrane to swell and make it easier to see and remove as shown in the video. Demembranate embryo in 100% HBSt by carefully grabbing near neural crest cells with both tweezers and pulling to form a hole as described in the dejellying step above.
7. Dip all tools into 70% EtOH and then into sterile HBSt before using.
8. Hold recipient embryo ventral side up with hair loop and pick a small slit down the midline of the embryo along the anterior-posterior axis with eyebrow microscalpel or tungsten microscalpel.
CAUTION: Be gentle. Do not slice through the entire embryo and be careful not to cause inner cells to spew out of embryo!
9. Place foil in the incision deeply enough so that it does not fall out but not so deeply that it cuts through the embryo. Observe development until heart forms.
10. Leave one embryo with only the incision but no foil, and one embryo uncut as controls.
11. While the high Ca2+ concentration of the HBSt enhances healing by saturating the Ca2+ binding sites of cadherin molecules, a high salt concentration also causes developmental abnormalities. Therefore, after embryos heal from surgery, gently replace 100% HBSt with 50%, then 20% HBSt. Change solution concentrations after 12 hrs.
12. Collect pictures and movies daily using a microscope equipped with a camera and recording cameras.
Graft technique
1. Follow previous protocol for preparation of microtools and operating dishes, dejellying, and demembranation of stage 15 recipient embryos (Figure 1 A, below) and stage 20 donor embryos (Figure 1 B).
2. Position donor embryo on lateral side and recipient embryo on dorsal side (neural crest-side down) exposing ventral side in separate wells of operating dish.
3. Hold donor embryo with hair loop instrument and use Tungsten microscalpel to remove small chunk of tissue from lateral, gill-forming surface.
CAUTION: Do not remove a chunk of neural tube. These have the tendency to adhere strongly to themselves and will therefore not heal well in the recipient embryo.
4. Hold recipient embryo ventral side up with hair loop and pick a small slit down the midline of the embryo with eyebrow microscalpel or tungsten microscalpel (Figure 2 A).
CAUTION: Be gentler than when cutting the donor gill chunk. Do not slice through the entire embryo and do not cause inner cells to spew out of embryo!
5. Imbed donor gill-forming piece in the incision on the recipient embryo. Pat down and ensure its position with eyebrow or tungsten microscalpel.
6. Leave one recipient embryo with only the incision but no graft, and one embryo uncut as controls.
7. Observe the development of the embryos until heart forms.
8. As described previously, the high Ca2+ concentration of the HBSt enhances healing by saturating the Ca2+ binding sites of cadherin molecules, but a high salt concentration also causes developmental abnormalities. Therefore, after embryos heal from surgery, gently replace 100% HBSt with 50%, then 20% HBSt. Change solution concentrations after 12 hrs.
9. Collect pictures and movies daily using a microscope equipped with a still camera and recording cameras.


Figure 1. Dejellied test embryos. (A) Stage 15, early neurula II recipient embryo. Clear vitelline envelope is swollen and visible after soaking in 100% HBSt. (B) Dejellied, demembranated, stage 20, late neurula IV donor embryo. Donor grafts were taken from the gill-forming region of a stage 20, late neurula IV, shown by arrow.


Figure 2. Recipient embryos. (A) Stage 16 recipient embryo with incisions down midline of ventral side. Actual incisions were larger than this photograph portrays. (B) Recipient embryo with graft in incision. Dark donor tissue sits in lighter area of incision.
Foil Procedure:
The results of the study indicate that development in the axolotl is under regulative control. Figure 1 shows a control and bisected axolotl embryos. The insicision of this particular embryo shows the bisection of the heart morphogenetic field. The movies show the result of the bisection of the heart morphogenetic field. It is clear that the bisected embryo has two hearts.
Figure 1. The bisected heart field of an axolotl embryo after three days (A). The control embryo after three days (B) Bisected embyo
Grafting Procedure:
Of the almost one hundred embryos used in the grafting procedure alone, only sixty-two survived the dejellying process. Twenty-one embryos were killed during the demembranating procedures. Twenty were in the early neurula II or III stage (stages 15 or 16) of development and appropriate for this experiment (Bordzilovskaya, 210). Eleven embryos were killed during the grafting surgery. These embryos generally had too large an incision made down the midline of the ventral side, which resulted in the spilling out of the light tan colored endo- and mesodermal cells (Figure 3). Only nine embryos were successfully grafted and set aside with the two control embryos
Forty-eight hours after surgery, most of the embryos had reached stage 27 and some of the embryos were moving by themselves (Figure 4). One embryo responded to touch by wiggling the anterior half its body back and forth. Four of the embryos had damage near the anterior end, consisting of an area where the dark ectodermal cells were missing or barely attached to the body and the lighter colored endo- and mesodermal cells were visible or hanging out the body (Figure 5A). Clumps of cells had fallen out of the embryos and were floating in the HBSt solution. After five days, seven of the nine test embryos had disintegrated into an unorganized mass of cells (Figure 5B). By day six, all embryos, including the controls, had died.
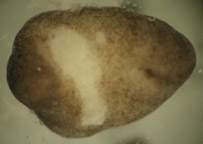
Figure 3. Surgically damaged recipient embryo. The incision was too large, resulting in the loss of light tan colored endo- and mesodermal cells. Embryos damaged in this way were ineligible for the experiment.

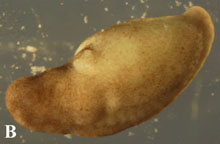
Figure 4. Post-operational development of test embryos. (A) 12 hours after operation, graft is still clearly discernable from host embryo tissue. (B) 48 hours after surgery, embryos had grown to stage 27. The graft is partially covered with host ectoderm cells and is becoming less prominent. (C) By 72 post-surgery, embryos had developed to stage 32 and the incision was barely visible.


Figure 5. (A) Injured stage 34 embryo four days post surgery. Embryos were found with injuries near the anterior head-forming region and often had lost some endo- or mesodermal cells as well. (B) Embryos six days post surgery. Embryos eventually disintegrated into masses of cells with no discernable organization.
The results of the foil procedure support the hypthesis that the heart morphogenetic fields in the axolotl are under regulative control. Morphogenetic fields are veryimportant in that they help determine how different organs in the axolotl develop (from the heart and gills to the limbs). The results of the study confirm this idea by showing that two hearts formed when an impermeable barrier was placed between the heart primordia. The movies show that the same axolotl had two hearts, one on each side of the body. After the bisection the two sides of themorphogenetic field were not in direct communication. As a result both heart primordia developed into a complete heart. If there had been no bisection, the heart field would have just developed into one heart.
No embryos survived to stage 37 with fully developed hearts in the grafting procedure. There are a number of possible reasons for the deaths of the embryos. Being caught in the wells or agar of the surgical dishes could have irreparably injured the embryos. Only one case was documented in which an embryo was left in a well and grew there until it got stuck. This embryo was partially damaged when it was removed from the well. Injuries to the head regions may have been due to the agar. To prevent further possible damage, the embryos were transferred to agarless petri dishes as soon as they appeared sufficiently healed from surgery. This terminated the instances of embryos being damaged by the agar.
If the embryos did not heal before they reached stage 29, they would begin to move and injure themselves, eventually causing themselves severe damage. Healing could have been disrupted if the grafts were composed of neural tissue. However, special care was taken to cut the graft from the non-neural, gill-forming ectoderm region on the lateral side of the donor embryo. Thus, while it is likely that the embryos did not heal from the surgery, it was presumably not due to the use of neural cells in the graft.
By the second post operational day, some embryos were beginning to move independently and to react to external stimuli. Some were moving and getting caught in different areas of agar. This could have disrupted or damaged the graft in some way. When they could not heal, the embryos died and their bodies degenerated to the pools of unorganized cells seen in Figure 5B (J. Cebra-Thomas, personal communication, 2004).
The failure of the embryos to develop fully functional hearts did not negate the value of this experiment. The methodology can be a useful tool for studying the types of regulation that occur in developing embryos. If the embryos had been moved to agarless dishes earlier, some of the injuries that may have contributed to the embryo death could have been avoided. Similarly, mistakes due to inexperience manipulating the microtools are very common and would be avoided if this experiment were repeated for practice. The incisions would be smaller, neater, and would therefore heal faster. Both of these changes would increase the survival rate and allow the embryos to develop far enough to display either regulative or autonomous development in the heart.